Battery Sizing guarantees that loads being supplied are adequately served for its designed autonomy time. Improper capacity sizing could jeopardize batteries used in applications such as Uninterruptible Power Supply (UPS), solar power systems, emergency lighting and telecommunications.
The calculations done on this post were based on the ampere-hour method for sizing battery capacity with reference to IEEE 485 and IEEE 1115. Also, it focuses only on lead-acid and nickel-cadmium (NiCd), which are two of the most common battery types used for UPS applications.
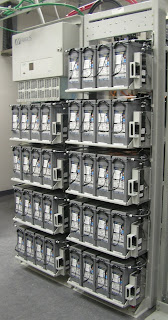 |
UPS Battery Bank |
The Battery Sizing involves these steps:
1. Identify the Battery Loads
Just like in UPS Sizing, the first step is to identify and select the loads that the battery will be supporting. This is dependent to the application of the battery, such as UPS systems. In this case, the battery load will be the same as that selected for the UPS.
2. Create the Load Profile
Refer to the UPS Sizing: Load Profile post for details on how to create a load profile and subsequently derive the design load and energy demand. IEEE refers to the load profile as the duty cycle. The resulting design energy demand in the example is 4020 VAh.
3. Select the Battery Type
IEEE suggested that the following factors should be considered in selecting the battery type:
Ø Application design life and expected life of cell
Ø Physical characteristics (dimensions, weight)
Ø Ambient temperature
Ø Charging characteristics
Ø Frequency and depth of discharge
Ø Ventilation requirements
Ø Maintenance requirements
Ø Seismic factors (shock and vibration)
Ø Cell orientation requirements
Subsequently, find the characteristics of the battery cells (generally from supplier data sheets), which includes the following:
Ø Battery cell capacities in Ampere-hour (Ah)
Ø Cell temperature
Ø Electrolyte density at full charge (for lead-acid batteries)
Ø Cell float voltage
Ø Cell end-of-discharge voltage (EODV)
For the example, the selected battery type is the valve-regulated lead acid (VRLA) battery.
4. Number of Cells in Series
The number of cells in a battery is computed to match the minimum and maximum voltage tolerances of the load. As a minimum, the battery at its EODV must be within the minimum voltage range of the load. Meanwhile, as a maximum, the battery at float voltage (or boost voltage) needs to be within the maximum voltage range of the load. The cell charging voltage depends on the type of charge cycle that is being used, e.g. float, boost, equalizing, etc, and the maximum value should be chosen.
The number of battery cells required to be connected in series must fall between the two following limits:
Nmin = Vdc*(1+Vmax) / Vc
Nmax = Vdc*(1-Vmin) / Veod
where:
Nmin = minimum number of battery cells
Nmax = maximum number of battery cells
Vdc = nominal battery voltage (V)
Vmin = minimum load voltage tolerance (%)
Vmax = maximum load voltage tolerance (%)
Veod = cell end of discharge voltage (V)
Vc = cell charging voltage (V)
As an example, the minimum and maximum load voltage tolerances are Vmin = 10% and Vmax = 10%, respectively. For the battery, nominal voltage is Vdc = 120V, end-of-discharge voltage is Veod = 1.8V/cell, and the cell charging voltage is Vc = 2.05V/cell. Then,
Nmax = 120(1+0.1) / 2.05
Nmax = 64.4 or 64 cells
Nmin = 120(1-0.1) / 1.8
Nmin = 60 cells
Use 62 cells in series (number of cells between 60 and 64).
5. Determine Battery Capacity
Other than the energy demand and battery nominal voltage, several other factors may affect the computation for battery capacity. These factors are discussed below:
Aging Factor (ka)
Battery performance decreases with increase in age. To ensure that the battery can meet capacity throughout its useful life, an aging factor is applied - generally 1.25. There are some exceptions - consult the supplier.
Temperature Correction Factor (kt)
Batteries are specified by a standard operating temperature of 25°C, a deviation from this value should be taken into account by applying a correction factor. The values can be gathered from the manufacturer. However, high temperatures reduce battery life irrespective of capacity and the correction factor is for capacity sizing only. Meaning, battery life can’t be increased by increasing capacity.
Capacity Rating Factor (kc)
This accounts for voltage depressions during battery discharge. IEEE 1115 Annex C suggests that for float charging applications, Kt = rated capacity in Ah / discharge current in Amps (for specified discharge time and EODV).
Taking the abovementioned factors into consideration, the minimum battery capacity required to carry the design load over the specified autonomy time shall be calculated as follows:
B = [Energy demand X (ka X kt X kc)] / (Vdc X kd)
where:
B = required battery size or capacity
ka = aging factor (%)
kt = temperature correction factor (%)
kc = capacity rating factor (%)
kd = depth of discharge (%)
Vdc = nominal battery voltage (V)
Select the next standard rating that exceeds the minimum Ah battery capacity.
For example, compute for the minimum battery capacity using the given energy demand of 4020 VAh from UPS Sizing: Load Profile, battery aging factor (ka) = 25%, temperature correction factor at 30 deg C (kt) = 0.956, capacity rating factor (kc) = 10% and depth of discharge (kd) = 80%.
B = [4020 VAh X (1.25 X 0.956 X 1.1)] / (120 V X 0.8)
B = 55.04 Ah
Use a VRLA battery with a 60 Ah capacity.
References:
IEEE 485-2003. Recommended Practice for Sizing Lead-Acid Batteries for Stationary Applications
IEEE 1115-2005. Recommended Practice for Sizing Nickel-Cadmium Batteries for Stationary Applications